Let’s Build a Global Power Grid
With a little DC wizardry and a lot of cash, we could swap power across continents
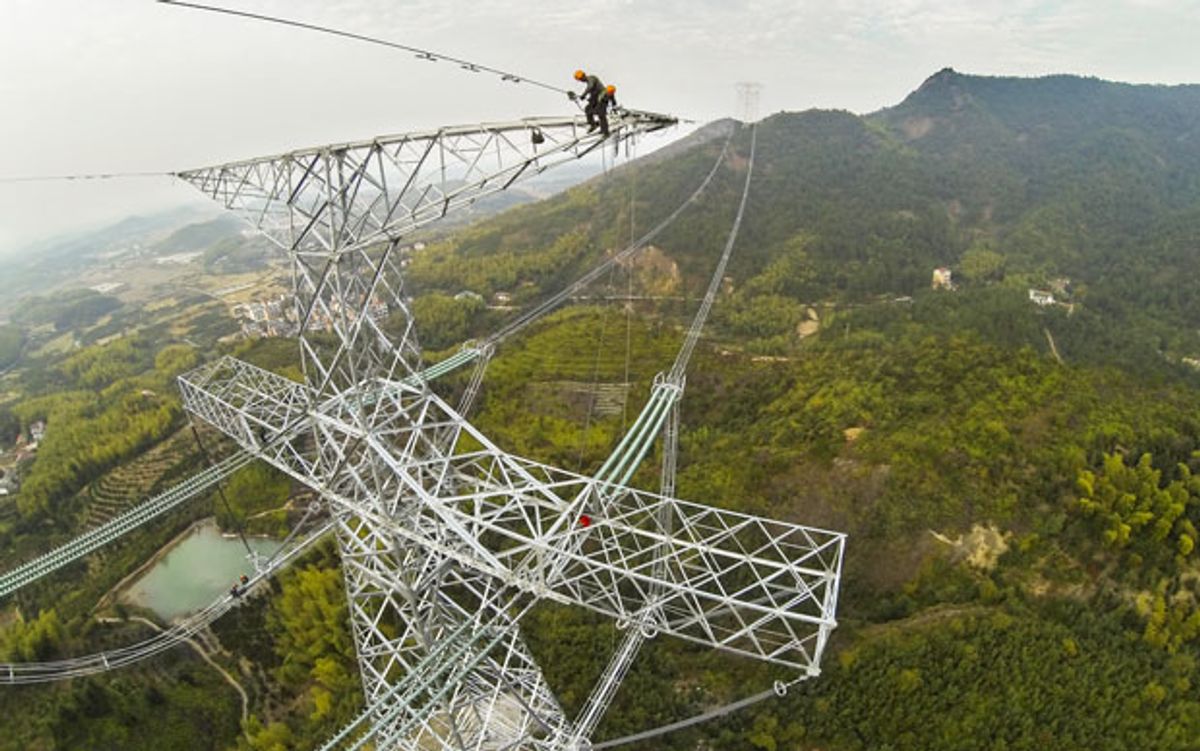
Reports abound of homeowners and businesses unplugging from the power grid and opting instead to generate and store their own electricity. Such grid defections may make sense in places where electricity rates are sky-high or service is spotty. But for just about everywhere else, it’s far more sensible to do the very opposite: interconnect regional electricity networks to form a globe-spanning supergrid.
What makes this idea so compelling are the major strains on today’s power grids: soaring energy demand in fast-growing megacities; rapid expansion of carbon-free but intermittent wind and solar power; and the ever-increasing need to secure grids against electronic and physical attacks. The smaller and more isolated a power network is, the more difficult it is to maintain the nearly instantaneous balance between electricity supply and demand.
But the technology now exists to transmit massive amounts of electricity over long distances without significant losses, thereby allowing operators to balance consumption and generation across an entire continent—or, potentially, the globe. If an outage occurs in one country, the sudden change in line voltage and frequency could trigger a generator thousands of kilometers away to compensate for the shortfall. Similarly, if the wind in a normally wind-dependent area dies, electricity from its neighbors could quickly fill in. Or if one region is experiencing heavy rainfall, hydroelectric dams there could capture the energy, to send elsewhere as needed. A supergrid would ensure that all or nearly all the electricity that’s generated would get consumed, thus avoiding such wasteful practices as paying wind-farm operators to curtail production or dumping energy that’s not immediately needed. (To be sure, storing excess energy would also help avoid such problems, but large-scale economical energy storage is still not widely available.)
In general, a global supergrid would allow power to be generated far from population centers. For instance, some of the world’s best sunlight can be found in the sparsely populated region south of Darwin, Australia, where it’s estimated that all of that country’s energy needs could be supplied from a solar farm the size of a cattle station. With an undersea link to Southeast Asia, that electricity could also be dispatched to countries like Indonesia, Papua New Guinea, and Singapore. And with a supergrid in place, operators could significantly scale back their spinning reserves—backup capacity that they can tap if demand spikes but in practice is rarely used.
So what would it take to build a global supergrid? Technologically, it would hinge on a globe-encircling network of high-voltage direct-current (HVDC) transmission systems, most of the components of which already exist. Beyond that, regional grid operators would need to agree on how to pay for such a network, establish rules for trading the electricity, and specify the technical codes and standards that will allow the supergrid to operate safely, reliably, and securely.
Constructed in 2002, the
Cross-Sound Cable consists of a pair of high-voltage DC transmission lines through Long Island sound that connects the electricity grids of New York and New England.Photo: ABB
The roots of the global supergrid stretch back to the dawn of the power industry, when the “war of the currents” raged between the era’s two great inventors: Thomas Edison and Nikola Tesla. In 1882, Edison demonstrated the first commercial electric power plant, which was based on direct current. But it was Tesla’s alternating current that would rule the day.
In 1895 Tesla’s dream of generating electricity from Niagara Falls was realized, and within a few years that energy was electrifying New York City, 700 kilometers away, thereby proving the superiority of the AC system. Well into the 20th century, the world’s power systems were based on AC.
The key to AC’s triumph was that power could be transformed to higher voltages by use of magnetic induction and then sent over long distances at low currents, minimizing the losses due to resistance; at the destination, the system would reduce the voltage for local distribution. At the time, there was no way to do the same with DC. But power engineers also knew that a DC system operating at high voltage would be superior to AC for the same task, because the amount of electricity lost during DC transmission would be far less than with AC.
How much less? Let’s say you’re transmitting a given amount of power by high-voltage DC: When you double the voltage, you need only half the current of a comparable AC system, thus reducing your line losses by a factor of four. You also need a lot less wire, because DC current penetrates the entire conductor of a power line, whereas AC current remains largely near the surface. Put another way, for the same conductor size, the effective resistance is greater with AC, and more power is lost as heat. In practice, that means the overall transmission infrastructure for AC far exceeds that for DC. To transmit 6,000 megawatts using a 765-kilovolt AC system, for instance, you’d need three separate single-circuit transmission lines, which would cut a right-of-way path about 180 meters wide. Compare that with an 800-kV DC system, which would require just one 80-meter-wide path.
HVDC also allows for the easy transfer of power between grids that are operating at different frequencies. The converters, cables, breakers, and other components for HVDC are more expensive than those for AC, so it only makes economic sense to use HVDC over distances of 500 km or more. But that break-even distance has continued to come down as the cost of DC components drops.
With these advantages in mind, power engineers experimented with DC transmission technology throughout the 20th century. The key building block for HVDC was and still is the converter, located at either end of an HVDC line. It serves to convert high-voltage AC to high-voltage DC and DC back to AC. Through the 1960s, such converters relied on mercury-arc valves, which were basically electronic switches that could only be turned on and not off, thereby limiting their functionality and resulting in substantial losses.
In the 1970s came the next advance in DC technology: water-cooled thyristors, a type of giant solid-state switch that can be turned on and off. The first ones were demonstrated in 1978 [PDF] at the Nelson River HVDC Transmission System, which sent power from hydroelectricity stations in northern Manitoba, Canada, to the country’s populated south.
Since then, HVDC has spread only modestly in North America, but it has taken off in other parts of the world, most notably Brazil, China, India, and Western Europe. Starting in the late 1990s, these newer HVDC deployments have relied on insulated gate bipolar transistors (IGBTs), which are specialized transistors that can be switched many times per cycle. In the latest IGBTs, the gates can open or close in less than a billionth of a second.
Today’s HVDC converters are known as voltage-source converters (VSCs). Although traditional converters continue to be used for higher-voltage, higher-capacity transmission, VSCs make it easier to integrate HVDC lines into existing networks. The VSC concept was first demonstrated [PDF] in March 1997 between Hellsjön and Grängesberg, Sweden, at a modest 3 MW and 10 kV. Five years later came the first sizable VSC installation: the Cross-Sound Cable project in Long Island Sound, between New York and Connecticut. That project had a moderately high rating of 330 MW, but conversion losses were also high, at 2.5 percent. With the latest VSCs such losses have been cut to just 1 percent.
What’s more, it’s now possible to have multiple terminals [PDF] along a single HVDC line, so that you can tap into the line at intermediate points, rather than just at the end. By moving beyond point-to-point HVDC transmission, you’ll be able to connect the lines into a mesh, which of course will be more complicated to control but potentially more robust.
First Steps to a Global Supergrid
Desertec:First proposed by a German-led consortium in 2009, the Desertec project aims to harvest solar power in the Mediterranean and other deserts of the world and use HVDC to transmit the electricity to population centers.
Medgrid: Similar to Desertec, the Medgrid plan calls for developing 20 gigawatts of solar power generation in North Africa, of which 5 GW would be exported to Europe. The Medgrid electricity network would become the backbone of the European supergrid.
China’s supergrid:To deliver solar and wind resources from the north and hydropower from the south to cities in the southeast, China has installed the most extensive network of high-voltage AC and HVDC in the world. It’s now expanding its transmission grid with 13 to 20 new HVDC lines.
Gobitec: Also modeled on Desertec, the Gobitec project would develop wind and solar photovoltaic systems in the Gobi Desert and deliver that power using an HVDC grid that would connect Irkutsk, Russia, in the north, with Shanghai and Seoul in the south and Tokyo in the east.
Southeast Asian supergrid:This supergrid proposal is envisioned as an undersea HVDC cable running from the northern coast of Australia along the Indonesian archipelago and up into the Philippines, Malaysia, and Indochina and then eventually into China, with the aim of exporting northern Australia’s abundant solar resources to Southeast Asia.
Asian supergrid:The proposed Asian supergrid would establish links between the electricity grids of China, Japan, Korea, Mongolia, and possibly Russia. An underpinning of the Asian supergrid is to enable free trade in electric power. Masayoshi Son, CEO of Japan’s Softbank, is said to be a key proponent of this plan.
Nordic grid: By 2030 Northern Europe is expected to see a substantial increase in renewable generation from wind and hydropower. While many of these countries are already interconnected to each other, further development of the Nordic Grid [PDF] will be needed to export the surplus to the rest of Europe and perhaps Russia.
North Sea Offshore Grid:Similar to the Nordic grid, the proposed North Sea Offshore Grid would harvest wind power generated in the North and Baltic Seas while opening broader markets for those resources.
IceLink: This is a recent incarnation of a 60-year-old idea to link Iceland’s power system with that of Europe through Scotland. Increasingly high electricity prices in Europe and more ambitious renewable energy targets have revived interest in the IceLink project.
Brazilian supergrid: To make use of hydropower in the country’s interior, Brazil is building a grid consisting of HVAC and 600-kilovolt HVDC, including the 2,385-kilometer-long Rio Madeira transmission link, the world’s longest.
Hydrogen-Electric Energy SuperGrid: Envisioned as a continent-wide underground HVDC transmission network, the Hydrogen-Electric Energy SuperGrid [PDF] would rely on hydrogen-producing advanced nuclear reactors. The transmission line, made from superconducting cable, would carry electricity as well as hydrogen to cool the cable. The hydrogen would also provide daytime energy storage for leveling energy consumption peaks. Excess hydrogen could be sold in local electricity markets or for commercial use.
Atlantic Wind Connection:This proposed offshore transmission line would span the mid-Atlantic region of the United States from New Jersey to Virginia to connect wind farms in federally designated wind energy areas.
What other technologies will the global supergrid need? One immediate need is fast-reacting, large-capacity circuit breakers that can handle short-circuit currents above 60 kiloamperes and respond to detected faults within milliseconds. Three years ago, the Swiss company ABB announced a hybrid electromechanical breaker that gets part of the way there. Earlier this year, Siemens announced that it had successfully tested 5-kA breakers for China’s Xiluodu-Jinhua HVDC line, which runs from Sichuan province to Zhejiang province. Alstom has also reported a circuit breaker prototype in this range. But much more work is needed to bring down the cost and size of these devices and boost their performance.
In the longer term, power cables made from high-temperature superconducting materials instead of copper or aluminum would greatly accelerate the deployment of the global supergrid because they can handle substantially higher power with essentially zero losses. Although they do need to be cooled to liquid-nitrogen temperatures—below 77 kelvins—the losses from refrigeration and other sources are less than half those of conventional AC and DC overhead transmission lines. And superconducting DC cable requires even smaller rights-of-way than does HVDC with traditional cables. Several projects have demonstrated superconducting cables over short distances, such as the recently installed 500-meter, 80-kV DC line on the Korean island of Jeju. At present, though, they’re still considerably more expensive than conventional cable.
Another promising area involves advanced power electronics made from materials other than silicon. Silicon’s abundance, low cost, simple processing, and room-temperature operation have made it the material of choice for power and other semiconductor devices, but research on novel materials such as silicon carbide and gallium nitride has shown real promise for the kind of power switching required in HVDC networks. These wide bandgap semiconductors can operate at higher temperatures than standard silicon devices do, and they support higher currents and higher voltages with less resistance. If they can be made commercially viable, wide bandgap devices would both reduce the cost and increase the functionality of HVDC converters.
If you were to build a global supergrid, where would you start? One obvious place would be China, which is now the world leader in the development and deployment of grid technology, in particular HVDC. The country has begun to exploit its massive potential solar and wind resources in the north and substantial hydropower potential in the south. To deliver that approximately 1,300 gigawatts of generation to population and industrial centers in the east and south, China has already installed the most extensive network of high-voltage AC and HVDC in the world, and over the next five years it plans to build another 13 to 20 ultra HVDC lines (in the 800-kV and 1100-kV range). Those upgrades have not come cheap: In 2014 China spent US $65 billion on such projects, and it is expected to sustain that level of spending over at least the next five years. The International Energy Agency estimates that China will need to spend more than $4 trillion from now until 2040 to overhaul the way it transmits and distributes electricity. Liangzhong Yao, vice president of renewable energy and smart-grid technologies at the China Electric Power Research Institute, has reported that his group is also looking at the feasibility of an intercontinental transmission grid to connect China with Europe, the Middle East, and North Africa.
Another logical starting point for a global supergrid is Europe, where the European Commission has been calling for a pan-Europe supergrid since 2008. The planning for such a grid is being led by the European Network of Transmission System Operators for Electricity (ENTSO-E), which represents 41 transmission system operators in 34 European countries.
The general idea is to build an HVDC grid that would connect these European countries with neighboring regions, including Kazakhstan, North Africa, and Turkey. A study by Gregor Czish of Kassel University, in Germany, found that with such a grid in place, Europe’s energy needs could be supplied largely by wind power, with only modest amounts of biomass generation as a supplement. An industry group called Friends of the Supergrid has been vocal in promoting the technology, regulation, and financing that would be needed to pull off such an ambitious plan.
French Connection: A recently completed HVDC link between France and Spain doubles the amount of electricity that can be exchanged between the two countries. Converter stations like the one shown here sit at each end of the line, converting high-voltage AC to DC and DC back to AC.Photo: Siemens
To date, a number of key European HVDC interconnections have been completed or reached advanced stages of planning, including the €10 billion project to connect Germany’s energy-rich north with its energy-starved south, two others to link Germany to Norway and Norway to Denmark, and a just-completed HVDC intertie between France and Spain.
To be sure, a global supergrid would require quite a bit more infrastructure: by my estimate, roughly 100,000 km of HVDC lines and 115 converter stations, based on planned projects and assuming redundancy in some areas. A few of these stations would be “superstations,” such as the one envisioned by the developers of the Tres Amigas project in New Mexico. Tres Amigas is intended to connect North America’s three primary grids (west, east, and Texas) and also provide some energy storage. The global grid will need something similar wherever large regional grids come together. (See “First Steps to a Global Supergrid” for a list of proposed supergrid projects in Asia, Europe, and other parts of the world.)
By far the greatest hurdle facing the global supergrid is how to pay for it. It’s hard to attach a firm number to such a vast and complicated undertaking, but proponents point out that its costs would be more than offset by its many advantages. In a 2013 paper in the journal Renewable Energy, Spyros Chatzivasileiadis, Damien Ernst, and Göran Andersson looked at previous studies of supergrids as well as completed projects to estimate what it would take to build an 800-kV, 3-GW undersea HVDC cable that’s 5,500 km long—about the length you’d need to connect New York City with Oporto, Portugal. (At present, 800 kV is significantly beyond what’s commercially available for submarine cables.) The authors concluded that the cable itself would cost from €1.15 million to €1.8 million per kilometer, and each terminal converter would cost about €300 million. Assuming thermal losses of 3 percent and a 40-year lifetime, among other things, the researchers estimated that transmitting electricity via such a cable would run between €0.0166 and €0.0251 (US $0.0189 and $0.0286) per delivered kilowatt-hour; the comparable figure for U.S. residential customers is about $0.011/kWh, which covers transmission but not power generation. The cost of the generated power with the HVDC supergrid would almost certainly be much lower than what’s available today because operators would be able to buy electricity from the least expensive source.
For the countries of the world to organize and fund a global supergrid, a strong international consensus for renewable (and perhaps nuclear) energy will need to form. This could be accelerated if a worldwide agreement could be reached on taxing greenhouse-gas emissions so as to create a financial incentive for shifting to carbon–free energy. Government funding will likely be needed to support the first segments of the global supergrid, but once those are in place, a carbon tax would help catalyze private-sector funding.
Beyond just the financing, governments and grid operators will need to agree on the rules for free trade in electricity. Electricity trading through a wholesale market, perhaps broken up into regions, would enable the kind of efficient power flows a global supergrid would offer.
A major collaborative planning effort will also be needed to bring together the existing grids and the planned regional supergrids. As noted earlier, China and Europe are well along in planning their HVDC networks. But in the United States, for example, transmission planning remains largely a state-level exercise, in part because states control land use and regulate investor–owned utilities. Such technical and logistical decisions as where to locate the supergrid’s points of connection, how to configure the HVDC grid, what voltages to use, and where and whether to use overhead or submarine systems will all need to be hammered out.
Last but certainly not least, all parties will need to agree on the technical specifications that will define the parameters of each transmission line, converter station, and generator so as to allow the global supergrid’s safe, reliable, and stable operation.
So, yes, a global supergrid will cost a great deal and no doubt take many decades to put in place. But obvious precedents for such an undertaking already exist in the international transportation and telecommunications sectors. And the alternative—doing nothing and continuing to rely heavily on fossil fuels and inefficient, disconnected power grids—will cost even more.
This article originally appeared in print as “A Globe-Spanning Supergrid.”
About the Author
Clark W. Gellings is a Fellow at the Electric Power Research Institute and an IEEE Life Fellow. This is his second article for IEEE Spectrum. His first, published in 1981, introduced the idea of demand-side management—a now common smart-grid practice that allows electricity customers to manage their own usage. “People thought it was heretical, but the idea eventually took hold,” Gellings says. “I hope that happens with this article, too.”