Smart Neural Stimulators Listen to the Body
Implanted devices sense vital statistics to deliver precisely tailored therapy
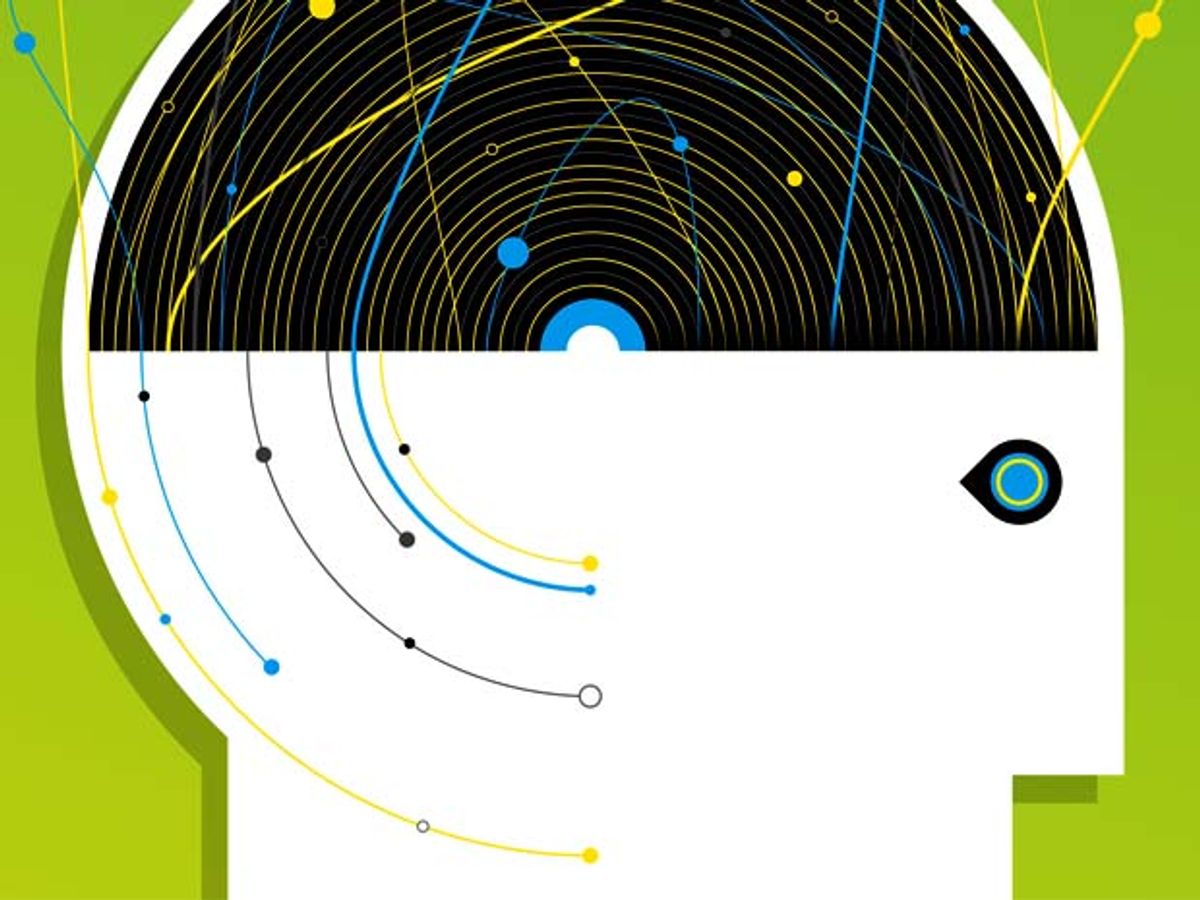
It’s an electrifying time to be in neuroscience. Using implanted devices that send pulses of electricity through the nervous system, physicians are learning how to influence the neural systems that control people’s bodies and minds. These devices give neurologists new ways to treat patients with a wide range of disorders, including epilepsy, chronic pain, depression, and Parkinson’s disease.
So far, these stimulators have been one-way devices that deliver a steady sequence of pulses to the nervous system but can’t react to changes in the patient’s body. Now, at last, medical device companies are coming out with dynamic neural stimulators that have a bit of “brain” themselves. These smart systems can detect changes in a physiological signal and then respond by delivering a therapy or adjusting the patient’s treatment in real time.
The three of us work for companies on this technological frontier, building devices that take advantage of developments in low-power implantable sensors and embedded signal processing. In this article we’ll describe three devices that respond to the flux of biology within the body. Because these devices rely on data related to the processes they influence, we call them “closed-loop” systems, but you could also call them the next step in a bionic model of medicine. In this new paradigm, engineered systems composed of chips, wires, and batteries can replace or supplement biological systems that malfunction.
An epileptic seizure starts with a storm of abnormal electrical activity in the brain. In the most common adult form of the disorder, this activity begins in one or two specific brain regions and can then spread to other parts of the brain, causing disturbances in movement, sensation, mood, and mental function. During a severe seizure, a person may have convulsions and lose consciousness. It’s a terrible and disruptive condition, and it’s fairly common: The World Health Organization estimates that about 50 million people around the world have epilepsy.
The majority of patients find their seizures can be controlled with antiepileptic drugs. For 30 to 40 percent of patients, however, these drugs don’t do the job. Neurosurgeons sometimes resort to cutting away the pieces of brain tissue where the seizures originate, but in the past decade or so they’ve had another alternative: They can implant neurostimulators. These stimulators send pulses of electricity through the nervous system in an attempt to prevent the electrical storms from commencing. An “open-loop” device, which stimulates the brain but can’t detect or respond to changing conditions, has been available since 1997. But the technology took a big step forward recently when NeuroPace, the Mountain View, Calif., company where one of us (Sun) works, invented a closed-loop device for epilepsy. This responsive neurostimulator (the RNS System), which received approval from the U.S. Food and Drug Administration [PDF] in 2013, both records information and delivers stimulation directly to the brain.
With the NeuroPace RNS System, the surgeon implants a slim device that houses the microprocessor and a battery into a small cavity in the skull. The device is connected to one or two leads that the surgeon carefully inserts into the brain; depending on where the seizures originate, the surgeon may place the leads on the surface of the cortex or implant them deep in the gray matter. Each lead has four electrodes that record the brain’s electrical activity and also deliver the stimulating pulses.
Here’s the beauty of this system: It works in the background, continuously sensing the electrical activity in the brain and delivering stimulation in response to specific patterns of activity that have been flagged by the physician. With just a few simple algorithms, doctors can configure the device to detect the electrical patterns—different in each patient—that indicate the onset of a seizure. These patterns are represented in the squiggly lines of an electrocorticogram and are composed of signals with unusual frequencies and amplitudes. When the device identifies one of these patterns, it triggers a stimulating pulse within tens of milliseconds. Doctors can also change the detection parameters over time if conditions change in the patient’s brain.
Patients may receive thousands of stimulations per day. However, with each burst lasting only a tenth of a second or so, the total stimulation time usually adds up to just a few minutes a day. Moreover, the brain doesn’t have pain receptors, so patients typically aren’t aware of the stimulation. This busy device doesn’t always stop the patient’s seizures altogether, but the NeuroPace clinical trial showed that patients implanted with the RNS System experienced an average 38 percent reduction in seizures within five months. And the frequency of seizures dropped further over the ensuing years, with the majority of patients experiencing a 50 percent or greater reduction in seizures by the two-year mark.
The technology has only just begun to help patients with epilepsy—256 people received implants during the clinical trials, and nearly 100 people have received commercial implants since then. But this modest start could be the beginning of a much broader research effort. Now that NeuroPace has figured out how to record and respond to the symptoms of one neurological disorder, the company may be able to build dynamic brain implants for others, perhaps helping people with movement disorders or mood disorders like depression.
There’s another way to head off an epileptic seizure with electricity. The Houston-based medical device company Cyberonics, where one of us (Morris) works, invented a device that stimulates the vagus nerve, which extends from the brain to the colon. Back in 1997, the company received FDA approval for the first version of the product, an open-loop vagus nerve stimulator (VNS).
For that basic VNS Therapy system, a surgeon implants a pulse generator in the chest and tunnels a lead up to the neck, where two spiral electrodes are wrapped around the vagus nerve. Typically, two weeks after the implantation procedure the pulse generator is programmed to deliver electrical stimulation pulses to the nerve, which sends them on to the brain. About 80,000 patients worldwide have received such implants.
The current open-loop system also allows on-demand stimulation, a freedom that comes in handy for those patients who can sometimes tell when they’re about to have seizures. Such premonitions are called auras, and they manifest themselves as illusions such as shimmering lights or strange smells. Thus warned, a patient can pick up the device’s external magnet, which looks something like a pager, and pass it over the implanted pulse generator to trigger a stimulation burst. In this way, patients who experience auras can prevent some seizures entirely and reduce the severity of many others.
Not all patients get auras, however, and not all can place the magnet over the pulse generator in time. Many people have seizures when they’re sleeping or just waking up, and some people’s seizures are accompanied by mental disturbances or unconsciousness. That’s why Cyberonics set out to create an automated version of the device—one that is always on alert, so the patient doesn’t have to be.
The company’s closed-loop AspireSR neurostimulator builds on the fact that seizures affect the neural circuits regulating the heartbeat; this means patients’ hearts begin to race as their seizures commence. So the new system’s implanted pulse generator includes a sensor that records an electrocardiogram (ECG), a readout of the heart’s electrical activity. This detection system has a big practical advantage: By looking for signs of seizures in the heart, this system treats epilepsy without invasive brain surgery.
While the sensor’s position in the chest is advantageous from a procedural perspective, electrical signals from the heart can be difficult to work with, as they can be obscured by signals generated by the muscles as the body moves. To compensate, the detection algorithm constantly monitors the quality of the signal; there are a number of checks that must be passed before the algorithm deems an electrical signal to be a valid heartbeat worth including in its calculations.
The next challenge is determining whether a heart is beating faster because of a seizure or from another cause entirely, like exercise, an argument, or an exciting football game. False positives aren’t a major concern, because the stimulation pulses are rarely perceptible to patients. At most, if the current is very high they might feel tingling in their throats or experience hoarseness. Too many false positives could tax the battery, however, which is intended to last four to seven years, depending on the programmed settings. So to detect a truly abnormal spike in heart rate, the algorithm compares the last few seconds of ECG signals with the last few minutes’ signals to calculate the relative change. If a person’s heart rate increases gradually as she starts to jog, for example, the threshold for triggering an electric pulse will go up. Likewise, as a person’s heart rate slowly decreases as he drifts off to sleep, the threshold will go down.
Cyberonics first conducted a study to compare the AspireSR’s detection of seizures against the current gold standard: visual inspection of graphs of the brain’s electrical activity (electroencephalograms, or EEGs). The system caught 80 percent of the seizures and did so quickly, in an average of 6 seconds. Subsequent clinical trials showed real therapeutic benefit. As with the brain stimulation system for epilepsy, the Cyberonics system doesn’t prevent all seizures, but in about 65 percent of patients the number of seizures was reduced by half.
The prior generation of VNS devices stimulated the vagus nerve in 30-second bursts about 11 times per hour. Cyberonics found in its clinical trial that when the new closed-loop device was set to its highest level of sensitivity, it added only a few extra stimulations to that total. Even if some of those additional pulses were the result of false positives, this seems acceptable. Vagus nerve stimulation doesn’t have the side effects associated with antiepileptic drugs, such as sluggishness and cognitive fog. In fact, patients using VNS therapy have reported feeling happier than usual and mentally sharper.
The AspireSR went on the market in Europe last year; it is awaiting FDA approval in the United States. Meanwhile, in the lab, engineers are continuing to refine the algorithms to detect more seizures with fewer false positives. They’re also considering adding a notification function, so that the first sign of a seizure would trigger an alert for a caregiver.
To be useful in a closed-loop system, a sensor needn’t always detect the electrical signals that flicker through the brain or heart; sensors can also pick up physiological signals such as those generated by movement and activity. Medtronic, where another of us (Denison) works, has developed one such system that uses a three-axis accelerometer as its sensor, bootstrapping off the technology in your smartphone.
People who suffer from chronic pain usually try drug regimens or surgery to alleviate their symptoms, but in some patients these approaches don’t work. So in the 1980s Medtronic came out with its first spinal cord stimulation (SCS) system, in which a pulse generator is implanted in the abdomen or above the buttock and leads are routed to the spinal canal inside the vertebrae. The system’s electrodes send an electric pulse up the nerve fibers that carry sensory information from the painful body part to the brain, generally producing a mild sensation called paresthesia—the tingling feeling of an arm or leg falling asleep. This stimulation to the brain is thought to interfere with the pain signal that the nerve fibers would otherwise transmit.
Today’s conventional SCS systems provide patients with remote controls to adjust stimulation if necessary and to maintain the desired levels of pain relief with limited side effects. For example, many patients find that changing body position alters the stimulator’s effect on their spinal cords, which can cause unwanted sensations. To understand this phenomenon, visualize a patient lying on his back; in that position, gravity pulls his spinal cord down closer to the electrodes, causing the current to activate more nerve fibers. The patient may then feel a jolt, or the tingling sensation may spread through more of his body. Conversely, if the patient lies on his stomach, the distance between the electrodes and the spinal cord increases, and because fewer nerve fibers receive the masking stimulation signal, the pain may return.
To address this issue, Medtronic set out to develop a closed-loop device that automatically adjusts stimulation intensity according to the patient’s position. While the best option might be a sensor that directly measures the distance between the electrode and the spinal cord using ultrasound or optical sensors, these technologies aren’t yet practical for a small implanted device.
A simple three-axis accelerometer, however, can provide an indirect gauge of distance: Its inertial measurements indicate the body’s position in real time, letting the device adjust stimulation as needed. For this purpose, Medtronic engineers adapted a type of microelectromechanical system accelerometer found in cellphones and other consumer products, which wouldn’t draw too much power and drain the device’s battery. The battery is rechargeable—the patient just holds an induction paddle over the implanted device—but it would be inconvenient if the patient had to recharge often.
In Medtronic’s adaptive SCS device, the implanted pulse generator contains both the accelerometer and a microprocessor, which runs an algorithm that classifies the person’s position and determines the appropriate stimulation. To calibrate the device initially, the patient assumes a series of postures (lying supine, prone, on the right and left sides, and standing up). The doctors can then map the data from each position to a stimulation level, with the aim of activating a constant volume of nerve tissue as the patient goes about the routines of daily life. The system also adapts over time by incorporating manual adjustments made by the patient into the automatic control algorithm.
Regulators in the United States and Europe approved this system, called the Restore Neurostimulator, a few years ago. The next generation of spinal cord stimulators from Medtronic may include other sensors as well—perhaps some that detect heart rate or signals from the central nervous system. As these implanted devices get smarter about what’s going on in a patient’s body, they should be able to deliver therapy that is more customized and precise.
As we see it, the goal of all these closed-loop systems is to let doctors take their expert knowledge—their ability to evaluate a patient’s condition and adjust therapy accordingly—and embed it in an implanted device. These dynamic systems have a number of potential benefits: They may react faster than current devices, provide more tailored therapy to individuals, and free up clinicians’ time.
These smart devices may have something to teach doctors as well. As the stimulators provide therapeutic effects, they also provide data about how physiological states relate to clinical outcomes. From this new information, scientists and engineers hope to learn more about how the nervous system works, how it is affected by disease, and how to design better treatments. Sometimes, it seems, the way to move forward in science is to follow a loop.
This article originally appeared in print as “Building a Bionic Nervous System.”
What’s it like to walk around with a stimulator in your brain? A woman with epilepsy describes her new life as a bionic woman.
About the Authors
Tim Denison is a technical fellow at the medical device company Medtronic, Felice Sun is a clinical science consultant at NeuroPace, and Milton Morris is former vice president of R&D and currently a consultant for Cyberonics. Their companies may be competitors, but the authors were glad for the opportunity to work together. “The challenges for individuals with neurological disorders are more important than the rivalries between companies,” says Denison.